There’s Something Essential in the Bank
Here are some techniques to remember for turning an aircraft.
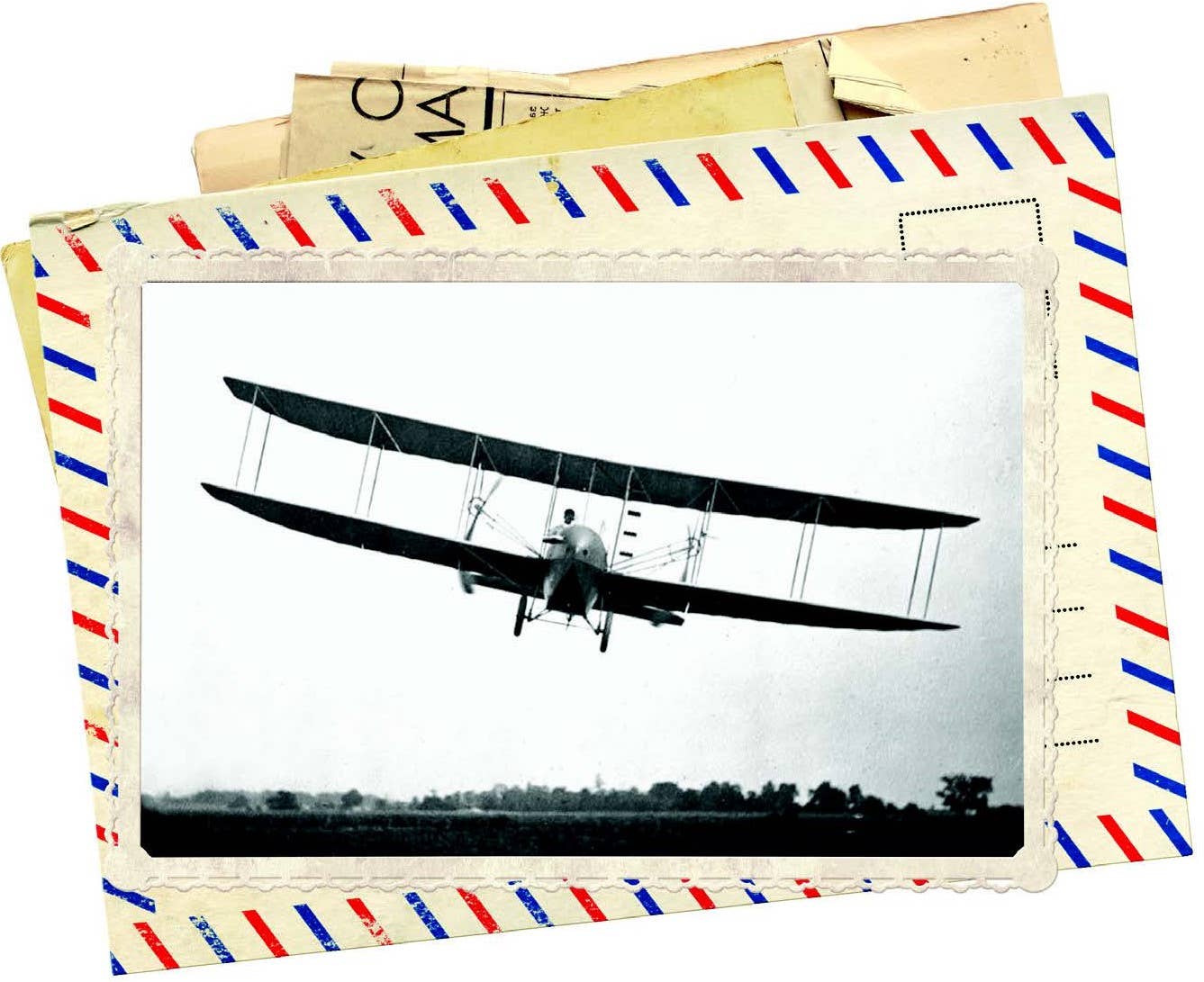
The ability to bank is essential to controlled flight. [Courtesy: Library of Congress; iStock]
The ability to bank is essential to controlled flight. Otto Lilienthal did it by shifting his weight, but for the much larger Wright Flyer the solution was to make one wing produce more lift than the other by twisting them in opposite directions.
“Wing warping,” as this approach was called, was satisfactory for very slow airplanes, but faster ones required more rigidity, and by around 1908 or 1909 the idea had arisen of replacing part of the trailing edge of a wing with a hinged, controllable flap. Actually, a prescient Englishman, Matthew Boulton, had patented the idea in 1868, when airplanes were still a thought experiment. His invention had been forgotten, however, by the time real airplanes came into being. Despite the early invention of the aileron, wing warping continued to be used, even on some fighters, as late as 1916.
If you're not already a subscriber, what are you waiting for? Subscribe today to get the issue as soon as it is released in either Print or Digital formats.
Subscribe NowThat a hinged trailing-edge flap would have the same effect as warping the entire wing is obvious to us, because we have seen it in action. But it cannot have been quite so obvious then. The evolution of airplanes in the United States suffered from the Wrights’ unfortunate attempt to establish a monopoly on flight by patenting the very concept of lateral control. Litigation over that ambitious claim held back aeronautical development in America for years while it raced ahead in Europe.
The function of an aileron, or any hinged trailing-edge surface, is commonly explained in ground school by simple analogy to, say, a door opened on a windy day. The wind hits the deflected surface of the aileron and pushes on it. If the aileron is deflected downward, the wind pushes it upward, and if it’s deflected upward, the wind pushes it downward.
This explanation, while intuitively appealing, fails to capture what is really happening. The majority of the lift generated by an unstalled airfoil is always concentrated near the front, and moving a trailing-edge flap up or down changes the flow conditions at the leading edge. An aileron deflected downward impedes air passing below the airfoil, and as a result, the dividing line between air passing below the airfoil and that passing above it moves aft. More air now passes over the top, the velocity of air rounding the leading edge increases, and the pressure there is correspondingly reduced. In other words, the lift change that results from deflecting the aileron is not confined to the aileron itself. It affects the entire area ahead of the aileron as well.
The effect of the aileron, like that of wing warping, amounts to a change in angle of attack. This understanding helps clarify what is happening in a steady-state roll. Why does an airplane with deflected ailerons settle at a steady-roll rate rather than roll faster and faster? It’s because the rotation reduces the angle of attack of the up-going wing and increases that of the down-going one. The change, which is opposed to the change caused by aileron deflection, is not uniform. It is greatest at the tip, where the rotational velocity of the wing, relative to the forward velocity of the airplane, is greatest. When the change of angle of attack due to rotation, integrated across the entire wing, is equal in magnitude to that resulting from deflection of the ailerons, the airplane is in equilibrium about its roll axis and rate of roll stops increasing.
Rate of roll is one of the “wow” numbers associated with a high-performance airplane. When we read that a T-38 or an A-4 rolls 720 degrees per second, we are amazed and wonder how the pilot knows which way is up. Arguably, roll acceleration—how quickly you get from zero to, say, 90 degrees of bank—might be more important in air combat maneuvering.
Roll rate is not a single number, however, as it increases with speed. Preferring a criterion that is independent of speed, engineers often refer to “peebee-over-toovee”—a (more or less) constant value represented by the ratio “pb/2V,” where “p” is the rate of roll in radians per second (a radian is 57.3 degrees), “b” is the wingspan, and “V” is the true airspeed (in the same units as the wingspan). Thus, for example, an airplane with a roll rate of 70 degrees per second (deg/sec), a wingspan of 35 feet, and a forward speed of 300 feet per second has a pb/2V of 0.071 radian.
In physical terms, that means that if the airplane flew past you while rolling, the path of its wingtip, in profile, would be at an angle of 0.071 x 57.3, or about 4 degrees to the flight path. That is called the “helix angle,” because the rotating wingtips form a double helix, like DNA.
The rolling helix angle is theoretically a constant for any given airplane, determined by wing planform, aileron design, and various other subtler factors. In principle it allows you to predict an airplane’s roll rate at any speed. Things don’t quite work out that way, however, because at high speed deflecting the ailerons makes the whole wing twist, counteracting the ailerons themselves, and cables stretch, preventing the ailerons from deflecting completely. Still, helix angle remains a convenient criterion, at the very least for setting a minimum acceptable standard for rolling performance.
A 1941 National Advisory Committee for Aeronautics report set that minimum value at 0.07 for transports and bombers and 0.09 for fighters. According to some published figures with which pilots who flew the airplanes are bound to disagree, the Spitfire Mk.V and FW-190 were fast-rolling airplanes, and the P-40 was not far behind. The FW-190 rolled 151 deg/sec at 226 knots, the Spitfire 150 at 176, and the P-40 134 at 315. The P-47 rolled a mere 71 deg/sec at 250 knots, the P-51B 98 at 260, the P-38 78 at 260. The corresponding pb/2V values are 0.118, 0.163, 0.082, 0.060, 0.072, and 0.084 respectively. The T-38 scores around 0.26.
Neither helix angle nor rolling acceleration fully expresses the quality of lateral control experienced by the pilot. That has more to do with effort, linearity of response, and presence or absence of “hysteresis,” or slop. Pilots used to single out the ailerons of Bellancas for praise, but pb/2V had nothing to do with it. It was really all about the ailerons’ smooth, frictionless, instantaneous response, low forces, and lack of free play.
The fact that pb/2V is theoretically constant for a given airplane has a couple of corollaries. One is that a larger span results in a lower roll rate. Another is that roll rate in degrees per second, taken alone, is misleading.
The Sopwith Camel, one of the deadliest fighters of World War I, rolled at a mere 40 degrees a second. But if you judged it by its pb/2V of 0.083, it was equal to the P-40 and superior to the P-47 and P-51.
This column first appeared in the March 2024/Issue 946 of FLYING’s print edition.


Subscribe to Our Newsletter
Get the latest FLYING stories delivered directly to your inbox