What We Can Learn from Ill-Fated Titan Submersible
Titan submersible’s service life limit unfortunately appears to have been only about a day or two.
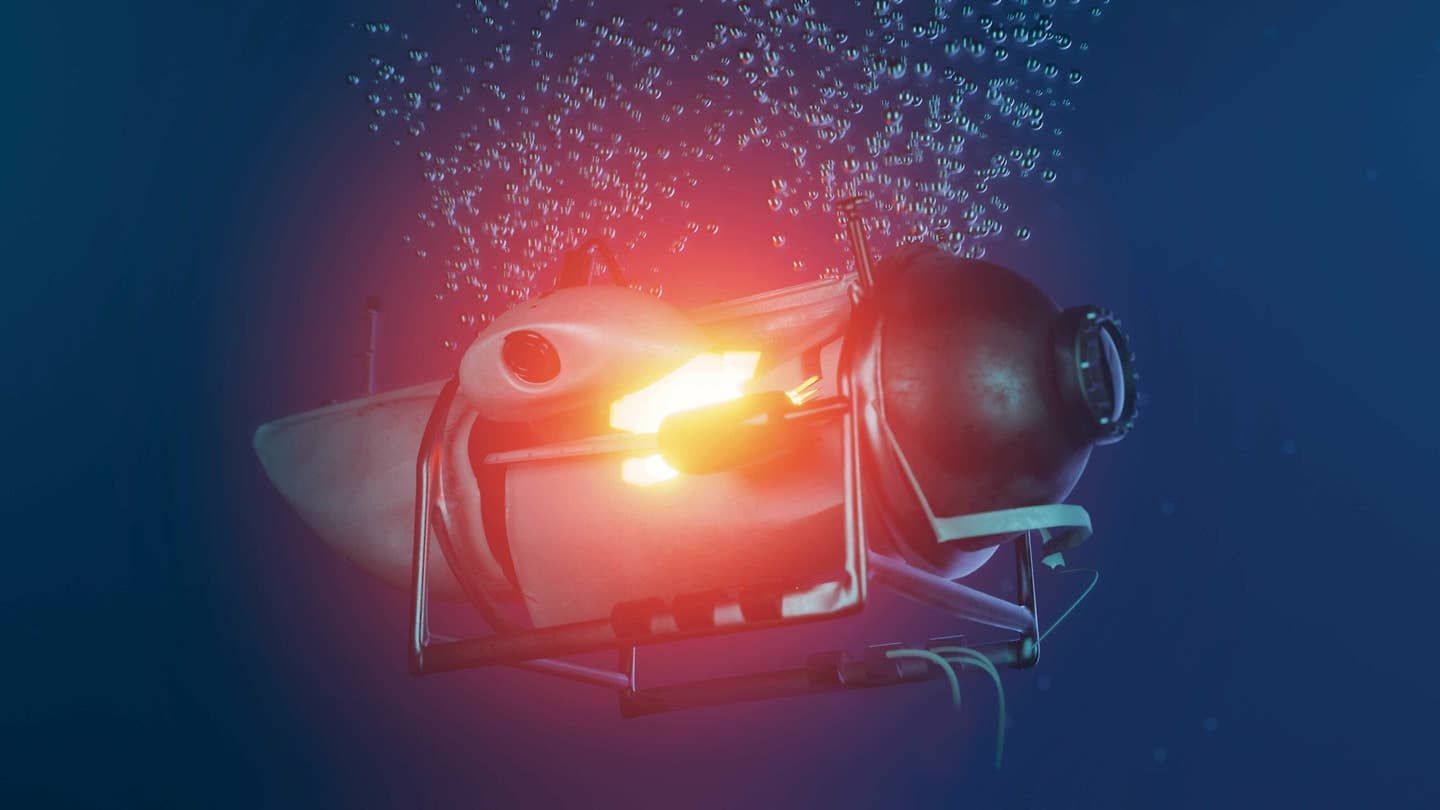
Until the investigation of the accident is completed, we
will not be certain why the ‘Titan’ submersible failed in June 2023. [iStock]
After the implosion of the submersible Titan in June, my friend Howard Morland wrote to me: “Cameron said it was ‘insidious’ the way composite carbon fiber materials ‘fail over time.’ This statement made me think of Melmoth 2. Any relevance?”
(Cameron being James Cameron, the film director and undersea explorer who was an early and outspoken critic of the Titan project; Melmoth 2 being my homebuilt airplane, whose wing structure consists mainly of carbon fiber composites. An unnamed third party in the drama is Stockton Rush, the designer of Titan and, like me, a self-taught amateur engineer.)
If you're not already a subscriber, what are you waiting for? Subscribe today to get the issue as soon as it is released in either Print or Digital formats.
Subscribe NowUntil the investigation of the accident is completed, we will not be certain why the submersible failed. But in case others wondered whether Cameron’s comments apply to carbon-fiber composites in aircraft…
Deep-sea submersibles are normally made of metal; carbon fiber construction was an innovation. Its high strength-to-weight ratio looked attractive for a craft that was expected to be very strong and yet able to float.
Carbon fibers are filaments two to four ten-thousandths of an inch in diameter—closer to spider web than human hair. They have a higher tensile strength than the strongest steel—around 500,000 pounds per square inch—and a compressive strength about a quarter of that.
You may wonder how such a slender filament can have compressive strength at all. Isn’t that like talking about the compressive strength of a rope? But if you take a sufficiently small segment of a filament, say only five or 10 times as long as it is thick, it is no longer a rope but a rod. And a rod can obviously support some amount of compressive load—that is, pressure applied to opposite ends.
We know from experience that if you push too hard on the ends of a long, skinny pole, it buckles. A 6-inch-long piece of uncooked spaghetti will bend outward and break if you press on its ends. A half-inch-long piece will hurt your fingers, but it won’t break.
You could make the longer strand carry a greater load if you tied it to a rigid support every half inch, so that it couldn’t buckle. This is the principle on which fine fibers in an epoxy matrix carry loads. Fibers are glued together to make a thick column within which each filament is continuously stabilized by all the others.
Compressive members are critical because if composite structures are going to fail, they are much more likely to do so in compression than in tension. Compressive components in airplanes, such as the upper portions of wing spars, are made of bundles of parallel fibers, not of the woven stuff found in fancy sporting goods. For the wing spars in Melmoth 2, I bonded multiple precured strips of unidirectional material that had been lab tested and found to fail in compression at 60,000 pounds per square inch. (Composites laid up in my garage and cured at room temperature do not attain the theoretical maximum strength of the material.) The way they would fail is significant: The surface fibers would pop out of the laminate. In other words, the force tending to bow the fibers outward exceeded the ability of the epoxy to hold them in.
This can happen within the laminate as well. Any imperfection—in epoxy, a tiny void, a speck of foreign material, a kink in a fiber bundle—can trigger “microbuckling” and minute delamination that grow with repeated loading. When Cameron spoke of carbon-fiber laminates failing insidiously over time, he was referring to this gradual accumulation of small cracks. Unlike cracks in metals, which usually appear on the surface and can be detected by visual or dye penetrant inspection, these are buried and detectable only when they have become quite large and then only by special testing equipment.
Titan’s pressure vessel, designed to withstand the 5,500-pounds-per-square-inch pressure where the Titanic wreckage rests, was an eight-foot long, 65-inch, carbon-fiber cylinder with hemispherical titanium end caps. At depth, each cap pressed on the cylinder’s ends with a force of around 18 million pounds. The aggregate pressure on the cylinder’s reportedly 5-inch-thick walls was more than 100 million pounds. If you assume a compressive strength of 125,000 psi and ideal fiber orientations, the hull would have had, when new, a factor of safety of around two. Because fiber orientations were probably not ideal, however, it was most likely lower than that, say 1.5 or even less.
Airplanes, too, use a safety factor of 1.5 over and above their highest anticipated aerodynamic loading. However, airplanes seldom, if ever, actually experience extreme loads, and if they do, it is only for very brief periods. A composite submersible, on the other hand, experiences extreme loading on every descent. Furthermore, it spends long periods at that loading, during which epoxy-to-fiber bonds can progressively deform and break. Indeed, people who had made previous dives in Rush’s composite submersibles—he designed several—report having heard cracking sounds, which must have concentrated their attention wonderfully.
An airplane undergoes a vast amount of strength and flight testing before being certificated and put on the market. The performance of its structure continues to be monitored, and the normally large number of examples in service gives the individual owner or passenger the comfort of knowing that if a problem occurs, it will probably happen to someone else first.
Titan was—and always would be—an experimental prototype. Notoriously, it was unclassed—the nautical analogue of uncertificated—because its creator, attempting to wrest the moral high ground from his critics, insisted that outside scrutiny puts a brake on the precious spirit of innovation.
Indeed, he was right. It does. Burt Rutan waxed eloquent on the unfair burden the required protection of desert tortoises placed upon the development of SpaceShipOne. But a brake is needed. Innovators are often people who are ambitious and headstrong, qualities that give them energy but may also blind them to the ethical implications of their actions. And this was not just innovation for its own sake. Rush was selling tickets.
Titan had made several previous deep dives and was evidently strong enough when it was new. But it apparently deteriorated with each prolonged imposition of enormous pressure. Composite airplanes, using similar materials and analogous construction techniques, are designed in the context of a huge accumulation of statistical data on loads encountered in flight—their character, strength, and duration—and on the behavior of composites in the flight environment. Composite airframes may have a service life limit, but none has yet been firmly established. Indications are that if one were established, it would be in the tens of thousands of hours.
Although the sample size falls short of statistical significance, Titan’s service life limit appears to have been more like a day or two.
This column first appeared in the September 2023/Issue 941 of FLYING’s print edition.


Subscribe to Our Newsletter
Get the latest FLYING stories delivered directly to your inbox