The Strut Rides Again
In its next concept aircraft, Boeing floats a classic way to reduce weight.
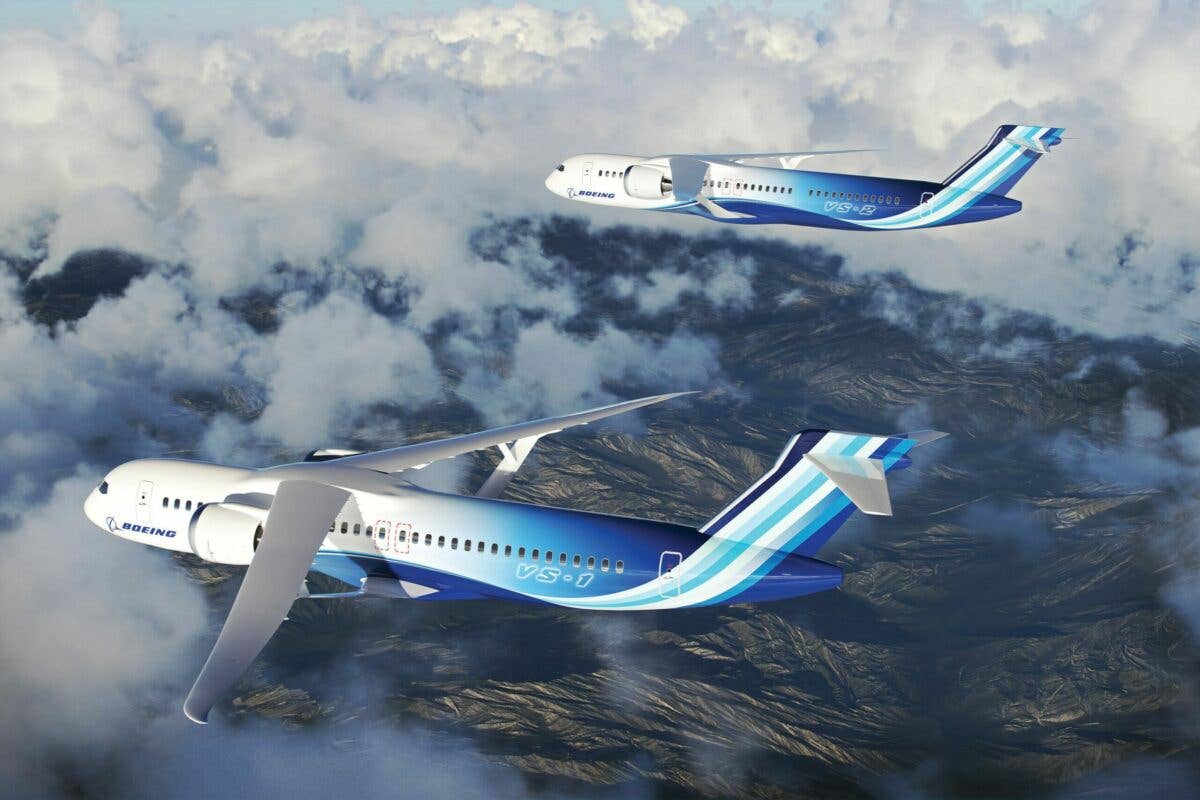
In January, NASA contracted with Boeing to build a proof-of-concept airplane called TTBW, for Transonic Truss-Braced Wing. [Courtesy: Boeing]
The Cessna 120, introduced in 1946, bequeathed its strut-braced wings to nearly all of its successors, making struts and single-engine Cessnas almost synonymous. It wasn’t always so. In the 1930s, Cessna built airplanes like the C-34, a clean radial-engine four-seater with a cantilever wing. The demise in 1954 of the C-34’s all-metal descendant, the 190/195, left the Cessna universe to strut-braced singles. So things remained until 1967, when cantilever wings appeared more or less simultaneously on the 177 Cardinal and the 210.
If you're not already a subscriber, what are you waiting for? Subscribe today to get the issue as soon as it is released in either Print or Digital formats.
Subscribe NowStruts and other external bracing were going out of use in fast monoplanes during the 1930s. Round wires generated as much drag as airfoils 10 times thicker. So-called “streamline tubing,” like that used for under-wing struts, produced more than 10 pounds of drag per square foot of frontal area at 100 knots. Since its pre-war designs had cantilever wings, why did Cessna revert to external bracing when it resumed business after the war? The likely answer lies in the interplay of power, weight, and drag.
The high drag of external bracing was never a secret. Fokker and Junkers had already recognized it a decade after Kitty Hawk and were building some cantilever monoplanes during World War I. But if reduced drag was one path to higher performance, reduced weight was another, and external bracing reduced the weight of wings. So long as airplanes didn’t go too fast, wings could be skinned with fabric or very light gauge aluminum; the only path to further reducing their weight was to make the spars lighter by adding a strut, which greatly alleviated loads in the otherwise highly-stressed inner portion of the spar.
Weight principally affects takeoff distance and rate of climb, whereas drag affects speed. For any airplane, there is going to be a crossover point at which the overall performance gained from a lighter spar is erased by the losses resulting from a strut.
That may not matter, however, in general aviation at least, because there is an even more important crossover point at which sex appeal outweighs rational design decisions. Cessna evidently reached that point with the 210 in 1967. Its struts looked stodgy compared with the cantilevers of its principal competitors, the Beechcraft Bonanza/ Debonair and the Piper Comanche; ergo, the 210 got a new wing, cantilever—and with laminar-flow airfoils to boot. The Cardinal, which was introduced at about the same time, also got a cantilever wing, which it hardly needed because with 150 horsepower it was, although a sweet airplane in many respects, grievously underpowered—a poor climber and quite slow. Logic would have given the Cardinal a strut, but it was intended to be a modern successor to the dowdy 172, and the cantilever wing fairly screamed: “Modern!”
That was more than half a century ago. Today the strut-braced wing is popping up again, this time in a most unlikely place: a proposed Mach 0.8 jet transport.
Theoretical study has been going on for more than a decade to establish the feasibility of greatly reducing the fuel consumption of airliners by increasing the aspect ratio of their wings. The idea isn’t new. In the 1950s, a French firm, Hurel-Dubois, produced strut-braced transports with sailplane-like aspect-ratio-20 wings. Respected aeronautical engineer Werner Pfenninger proposed a transonic airliner on the principle in 1975.
For a given wing loading and speed, induced drag—the drag due to lift—is inversely proportional to the aspect ratio. At the altitudes and speeds at which airliners fly, induced drag can be as much as half of the total drag of the airplane. Thus, doubling the aspect ratio of the wing could, in principle, reduce the total drag and fuel consumption by a quarter.
These elementary facts are not lost on Boeing, Airbus et al. But there’s a hitch. A higher aspect ratio means greater wing root stresses and hence greater structural weight. Current designs, products of decades of computer-aided refinement, already represent optimal compromises between aspect ratio and weight.
Re-enter, with fanfare, the lowly strut.
If you put the fuselage under the wing rather than on top of it and add a Cessna-style strut around mid-span, you can not only greatly reduce the structural weight of the inner part of the wing but also reduce the chord and thickness of the entire wing—both changes reduce drag—and increase its span. In other words, the strut allows you to increase the aspect ratio without a weight penalty, and the induced drag reduction more than pays for the drag of the strut and the various junctions it adds. You can even afford to add a third member, a short “jury strut” running from the main strut to the wing, to further reduce structural weight.
In January, NASA contracted with Boeing to build a proof-of-concept airplane called TTBW, for Transonic Truss-Braced Wing. The project, budgeted at over a billion dollars, will mate the somewhat shortened fuselage and the empennage of a McDonnell-Douglas MD-90 to a thin, short-chord wing with half again the span of an equivalent cantilever-wing transport.
There will be challenges. Elasticity and possibly flutter are liabilities of a very long, thin swept wing; active controls that react instantly to changing flow conditions might be a solution. Diminished fuel volume is another disadvantage; some fuel may have to be carried in the fuselage. Yet another is a lack of space at airports; an airplane of abnormally long span would have to fold its wingtips. (The Boeing 777X has folding tips that reduce its span by 22 feet; the feature is thought to entail a weight penalty of around 3,000 pounds.)
Illustrations accompanying the announcement of the Boeing contract show an MD-90 airframe with a very long, narrow high wing. The high wing provides plenty of ground clearance for big fat engines—good news if it’s a 737 replacement—but no place to mount landing gear. The artist has graciously omitted the bulging pods that will likely be required for that mundane purpose.
Both NASA and airframe manufacturers like to publicize futuristic studies like this from time to time. The last Next Big Thing was the BWB or Blended Wing-Body, which was preceded by Boeing’s improbable Sonic Cruiser, a Mach 0.95 aircraft with a canard strongly resembling the hapless Beech Starship. The cycle reminds me of the “dream cars” that General Motors used to cook up in the 1950s for its annual motoramas.
The interval between a novel conception and actual production is nicknamed the “valley of death.” The phrase comes from a Tennyson poem, “The Charge of the Light Brigade,” about a gallant but suicidal British cavalry charge against overwhelming Russian artillery in the Crimean War in 1854. The Sonic Cruiser quietly expired without flying in any form. The BWB got as far as a large RC model. The TTBW will become a full-scale test article. Let’s see if it fares any better than the Light Brigade.
This article was originally published in the April 2023, Issue 936 of FLYING.


Subscribe to Our Newsletter
Get the latest FLYING stories delivered directly to your inbox